IF YOU EVER WANT TO TAKE A HOUSE ON MARS
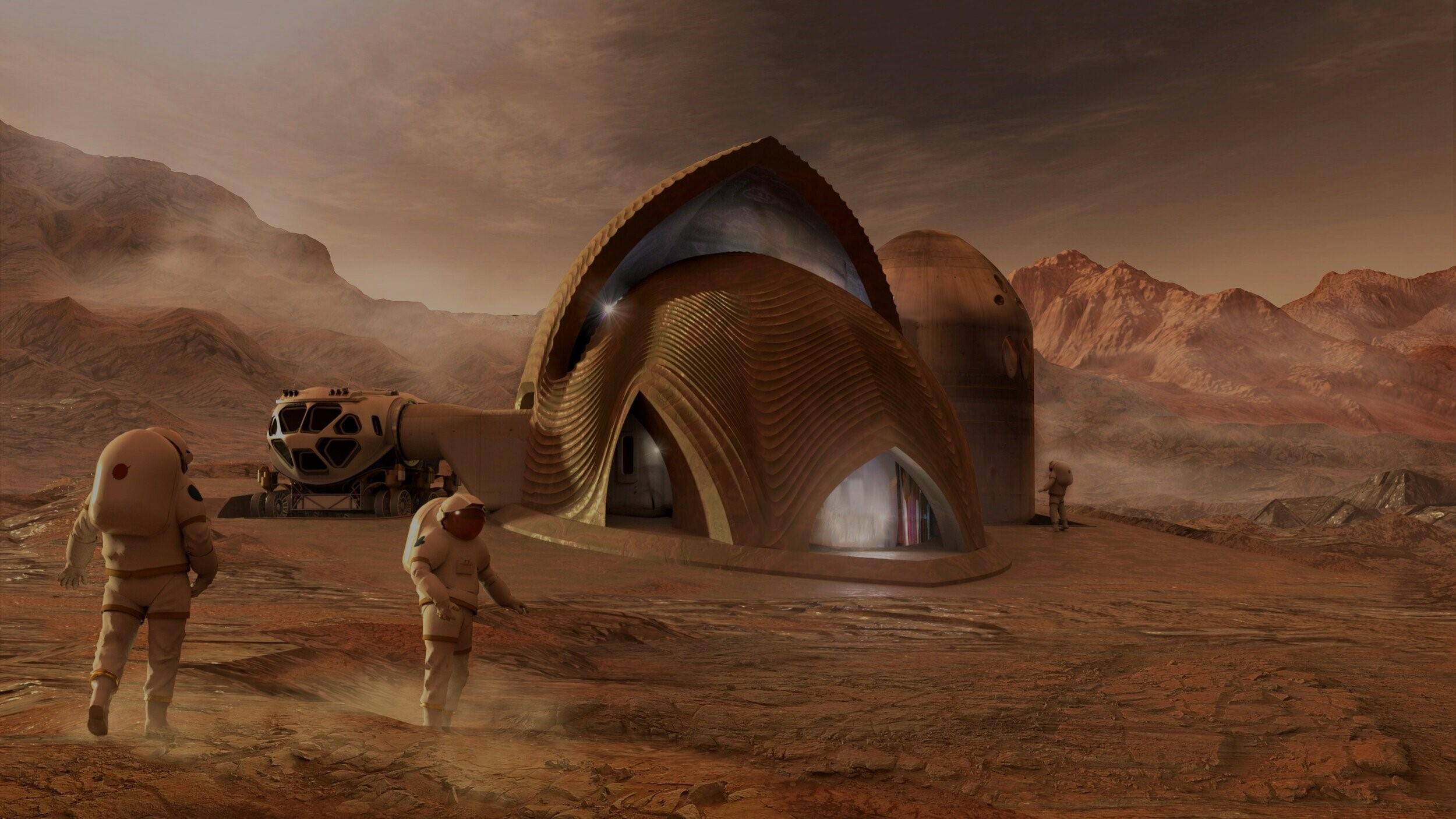
Man has always been a curious being, and his interest in exploring and understanding the universe around him thousands of years ago drove his gaze to the heavens. But today, we do not just observe celestial bodies with interest and admiration, but with the desire to conquer them. The colonisation of other planets has become one of the most interesting and pressing issues of our time. After many decades of space exploration and fantastic visions of human expansion in the universe, we are now on the threshold of reality, ready to realise the dream of colonising other worlds.
Almost 50 years after the last time an astronaut set foot on the Moon’s surface, NASA, in collaboration with the European Space Agency (ESA), the Japan Aerospace Exploration Agency (JAXA), the Canadian Space Agency (CSA) and a number of private companies, launched the Artemis mission with the aim of not only revisiting the Moon, but of creating the first human outpost beyond Earth’s orbit[1] .
The Artemis mission has the task of ensuring a long-term human and robotic presence on the Moon and its orbit and, if successful, to be an intermediate step before sending astronauts to Mars[2] . To do so, NASA will use SLS (Space Launch System), a space launch system in the form of a super-heavy modular rocket. It is the most powerful rocket created by mankind, capable of sending the Orion spacecraft, four astronauts and a large payload directly to the Moon in a single mission[3] .
Given the scope of the project, the mission is divided into phases. The first unmanned launch took place in November 2022 and Artemis I successfully returned to Earth in December 2022, after almost a month of flight over the Moon, demonstrating the capabilities of the Orion capsule, its service module and the giant SLS rocket[4] .
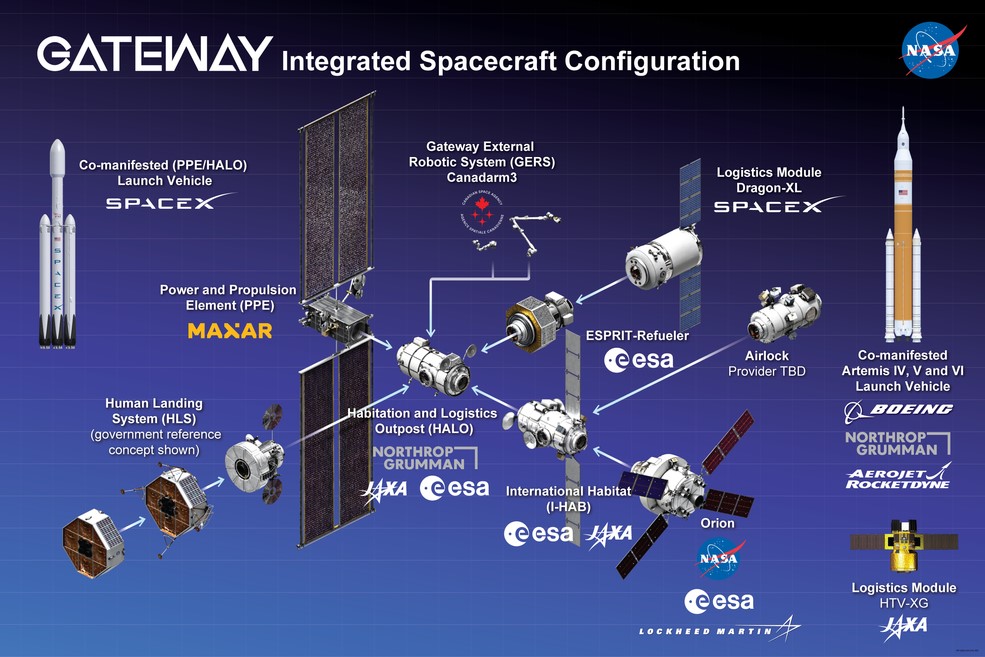
A complete overview of Gateway, including elements of international partners. Built with commercial and international partners, Gateway is crucial for sustainable lunar exploration and will serve as a model for future Mars missions[5]
NASA’s plan foresees the next launch of Artemis II with four astronauts on board in 2024, with the aim of circling the Moon. This will be followed by Artemis III in 2025, which will land on the Moon and remain there for about a week[6] , and the Artemis IV and V missions in 2027 and 2028, which will deliver Gateway, a small, human-operated space station in orbit around the Moon, in two arrivals[7] .
Behind all this action, there are many questions about habitability and human sustenance at such a distance from Earth, since the Moon is not the most suitable place to live and is about a thousand times further away than the International Space Station. The search for an alternative to utilise resources on site continues.
A home on another planet
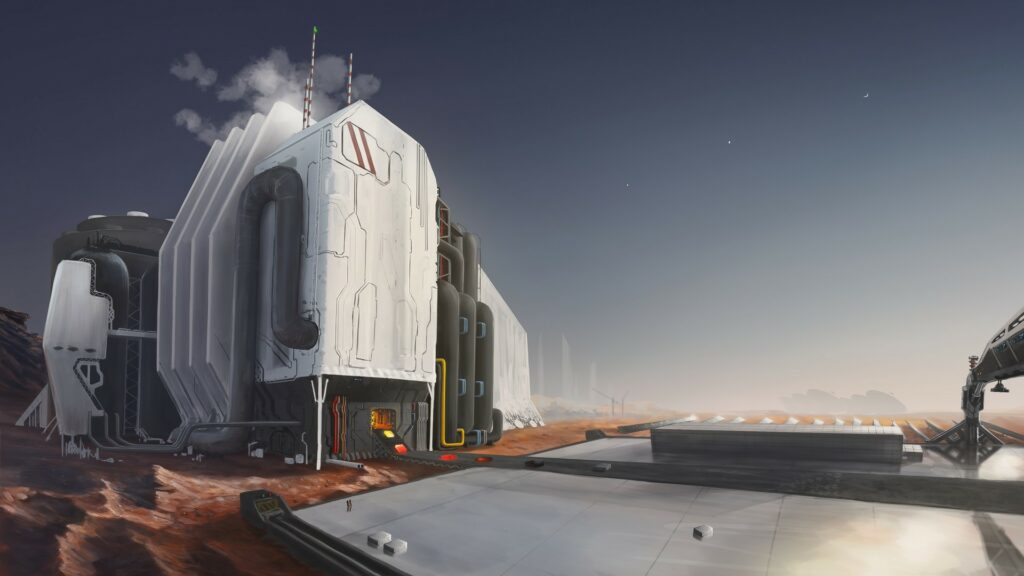
The project for a steel mill on Mars[8]
So far, sealed living quarters have been designed for the lunar space station, in which astronauts will be able to live and conduct research while visiting the lunar outpost. The accommodation module is based on the spacecraft currently used to transport cargo to the International Space Station[9] . As for the astronauts’ accommodation base on our satellite, NASA has awarded a $57.2 million contract to Icon, Texas, USA, to develop the technology needed to build roads, tracks and possibly houses on the Moon using 3D printing and lunar soil as material[10] .
As part of NASA’s NextSTEP programme, other companies are proposing alternatives. Lockheed Martin is developing inflatable habitat concepts that offer the possibility of building space objects with less weight, more volume and mobility, and lower installation costs than classical solid-wall structures[11] .
In the context of the colonisation of other planets, the term ‘in situ resource utilisation’ (ISRU) has emerged, i.e. the practice of collecting, processing, storing and utilising materials found or produced on other astronomical objects (Moon, Mars, asteroids, etc.) to replace materials that would otherwise be brought from Earth[12] . This direction is actively developing in the context of research into the properties of lunar and Martian soil and their suitability as construction materials. Scientists have shown that lunar and Martian soil can be turned into geopolymer concrete, which on our planet is an excellent environmental alternative to traditional cement[13] . But it is worth emphasising that the research was conducted on modelled lunar and Martian soil[14] .
Scientific challenges sometimes give rise to non-trivial solutions. British scientists have created a new material stronger than concrete, based on potato starch, extraterrestrial dust and salt[15] . This experiment is an improved version of the team’s previous work, in which they used the blood and urine of astronauts as a binding agent. The disadvantage of this method was the constant need for blood. In the new version of the study, starch is already used as food for astronauts and the salt, magnesium chloride, can be obtained from the surface of Mars or from astronauts’ tears[16] . In fact, in space, astronauts’ tears are a much more readily available material than their blood.
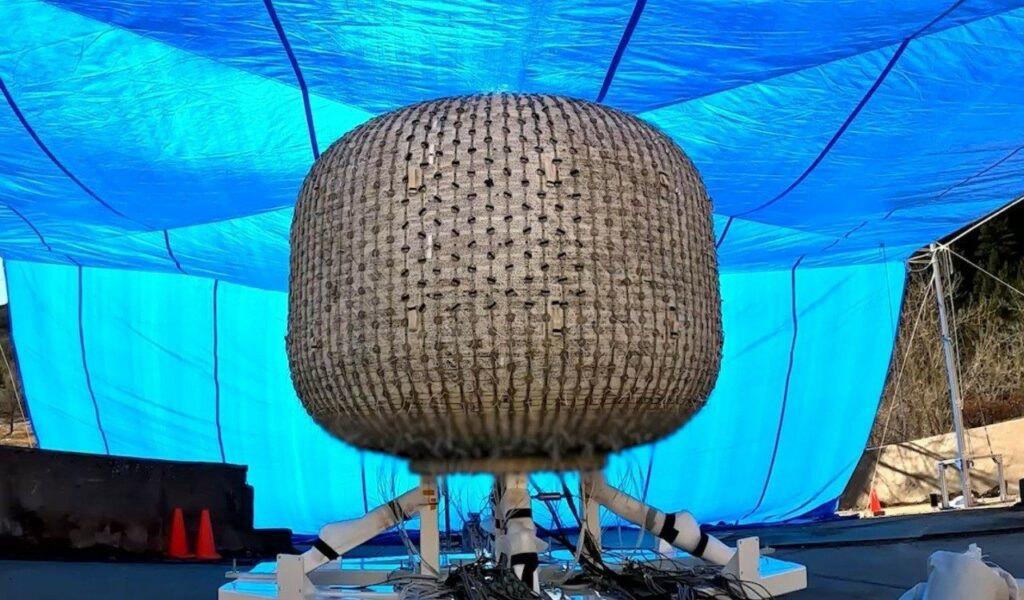
Lockheed Martin’s inflatable structure rupture test [17]
Any habitat, no matter what it looks like, needs electricity to function. The lack of atmosphere, in this case, plays a positive role and makes solar panels one of the most obvious and priority solutions[18] . A possible option could be an 18-metre-high vertical solar panel positioned on the Moon’s South Pole. The vertical position is dictated by the position of the Sun, which barely peeps over the horizon in this region. The panels would be connected by cables several kilometres long and attached to vehicles that could transport them to different locations[19] .
Eat, drink, breathe… colonise

Graphic illustration of mobile solar cells[20]
On the International Space Station, the issue of air and water is organised as follows: oxygen is produced by electrolysis, when an electric current is passed through the water and the liquid breaks down into oxygen and hydrogen molecules. The system has been in operation since the creation of the station. Approximately 1 litre of water is needed to provide one person with a daily dose of oxygen. There are oxygen cylinders on board for emergencies. Hydrogen is not used on the ISS and is released into space. Water, on the other hand, is occasionally delivered on cargo ships and is reused several times, thanks to purification systems. Approximately 93% of the liquids used or expelled, such as washing water, crew waste (urine) and excess moisture in the air, are collected, filtered and reused[21] . But let us not forget that the International Space Station is at an average altitude of 420 kilometres. It can take astronauts between 4 hours and 3 days to reach it, depending on the spacecraft used and the mission parameters[22] .
The duration and range of human space missions are about to increase rapidly, and life support systems are subject to high requirements and desirable autonomy. To meet the basic human need to breathe, NASA is already testing a high-tech system aboard the space station that will remove carbon dioxide (CO2 ) and moisture from inside Orion using an amine-based chemical combined with the vacuum of space. The Amine Swingbed system is designed to provide safe air for the crew to breathe and to control condensation and protect sensitive equipment[23] .
Air is obviously a life-supporting element for human life, but it is also a key component of rocket fuel. With the prospect of a trip to Mars, in addition to the propellant itself, the astronauts will have to carry twice their weight in oxygen to burn, otherwise it could be a ‘one-way trip’. For example, getting four astronauts from Mars back to Earth would require about 7 metric tonnes of rocket fuel and 25 metric tonnes of oxygen, not counting the oxygen needed to breathe. Therefore, the task of producing oxygen ‘locally’ is very challenging for scientists[24] .
In February 2021, NASA’s Perseverance rover landed on the Red Planet and attached to it was a block the size of a toaster MOXIE, which stands for ‘Mars Oxygen Resource Utilisation Experiment’, whose task was to produce oxygen on Mars. A molecule of carbon dioxide in the thin atmosphere of Mars is composed of one carbon atom and two oxygen atoms, and MOXIE simply extracted the oxygen molecules under the influence of high temperatures, around 800 degrees Celsius[25] . On 6 September 2023, the MOXIE mission was completed and managed to produce a total of 122 grams of Martian oxygen – about as much as a small dog inhales in 10 hours[26] .
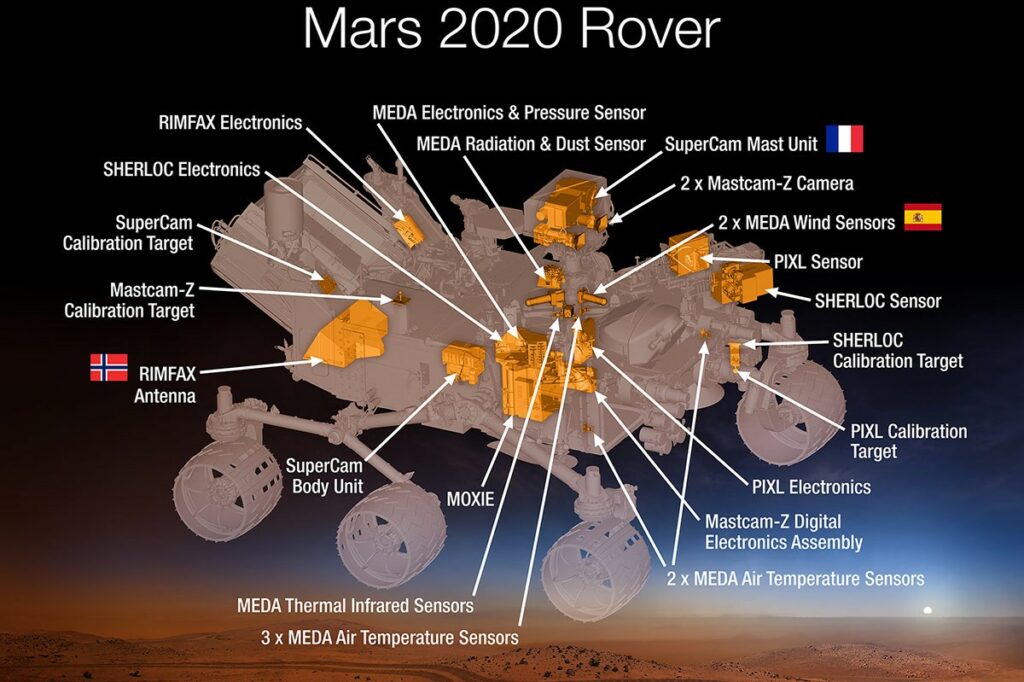
The single parts of the MOXIE vehicle[27]
The production and recycling of oxygen, carbon dioxide (CO2 ) and fuel are crucial for colonisation plans, as replenishing resources will be difficult and expensive or impossible. As part of the transition to clean energy on Earth, photoelectrochemical (PEC) devices are being investigated that can produce hydrogen and carbon-based fuels using sunlight from CO2 . They essentially replicate artificial photosynthesis like plants, but under the influence of electromagnetic radiation Their monolithic design and extreme dependence on solar energy make them interesting for space applications[28] .
A new ESA-sponsored study by scientists from the UK and Europe modelled the theoretical feasibility of these devices based on the expected environmental conditions on the Moon and Mars[29] . In particular, they studied the effect of lunar dust combined with solar wind causing electrostatic charges in the resulting dust and the frequent dust storms on Mars on the performance of the devices. Self-cleaning coatings have been proposed as a solution to the problem.
Reducing solar irradiation was also considered, and one of the technologies that could be used in solar-powered oxygen and fuel production devices on the Moon and Mars are solar concentrators, which provide devices with higher performance and greater power density. Thanks to this machine, scientists have shown that photoelectrochemical devices are a promising option for terrestrial, lunar and Martian environments when investigating the realistic efficiency of converting solar energy into chemical energy over the long term; however, from both an experimental and theoretical point of view, challenges and questions remain regarding the application of the devices on the Moon and Mars, because it does not take into account all the environmental challenges that a device faces in space, such as cosmic radiation, extreme radiation and other environmental problems[30] .
The south pole of the Moon, which is not even visible from Earth, attracts the attention of researchers. Due to the inclination of our satellite, the Sun is always around the horizon and at the pole only the high peaks are illuminated, while the low areas are in the so-called constant shadow regions. The temperature in this region can drop as low as -250 degrees Celsius, colder than that of Pluto, while the part illuminated by the Sun warms up to 120 degrees Celsius (due to the lack of atmosphere). Water molecules that enter this region of darkness are immediately frozen, with no possibility of evaporation, fall to the surface and mix with the lunar soil. This process leads to the formation of large deposits of water ice and makes this region extremely interesting from the point of view of the possibility of extracting water resources on the Moon[31] .
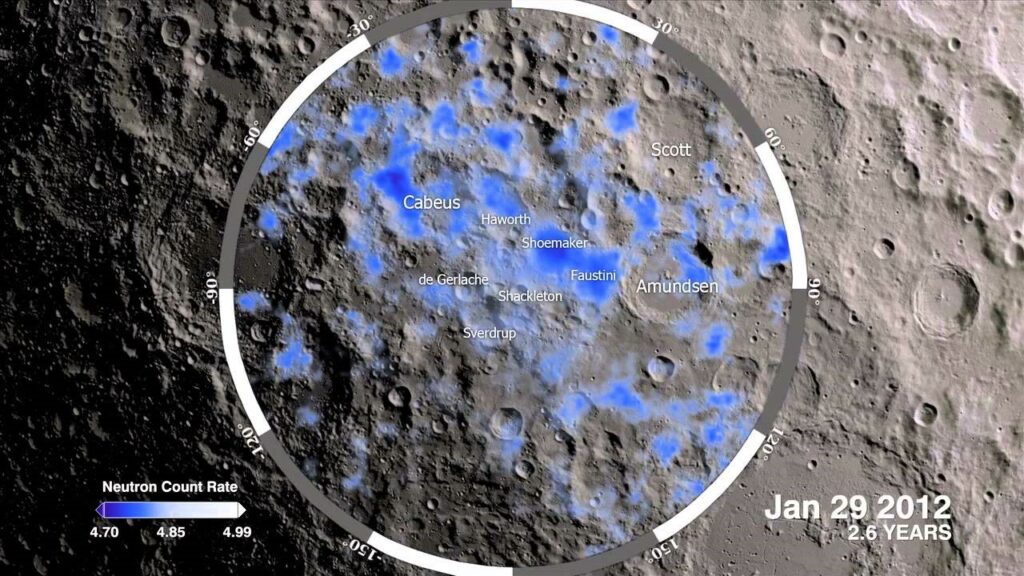
Possible ice deposits on the Moon[32]
Although visually the Moon looks like a dry ball of dust, research confirms that there is more water on our planet’s satellite than previously thought. The findings come after analysing impact glass in lunar rock samples collected by a Chinese rover during the Chang’e-5 mission. According to conservative estimates, up to 297.6 billion tonnes of water could be stored in the molten mineral remains that evaporated from the lunar surface due to the meteorite impact[33] .
While scientists are busy assessing the Moon’s water resources here on Earth, NASA’s Volatiles Investigating Polar Exploration Rover (VIPER) is on a mission to map water resources at the Moon’s south pole. The rover, which is the size of a golf-car and solar-powered, will use various scientific instruments and a 1-metre diameter drill to collect samples at precise locations chosen by experts and analyse them on site[34] . The researchers speculate that the water ice could be turned into drinking water and rocket fuel[35] . The VIPER was supposed to be launched in December 2022, but was postponed. It will be the first rover equipped with beacons and will have to visit places that are always in shadow and cover 20 kilometres in 100 days[36] .
But even if the source of the water matters little, whether it is extracted from ice or brought along, the important thing is that it is immediately usable. A project called BIOWYSE, led by the European Commission, hoped to find a solution to the problem of storing water for long periods of time, i.e. to control its contamination by micro-organisms, to disinfect it with ultraviolet light instead of chemicals if necessary, and to provide ‘in a cup’ fully usable drinking water. The prototype is a machine about one metre long, but with the prospect of creating a smaller sample for use in space. Scientists believe that the system itself could be useful for future missions, where water could remain unconsumed for months until the arrival of astronauts[37] .
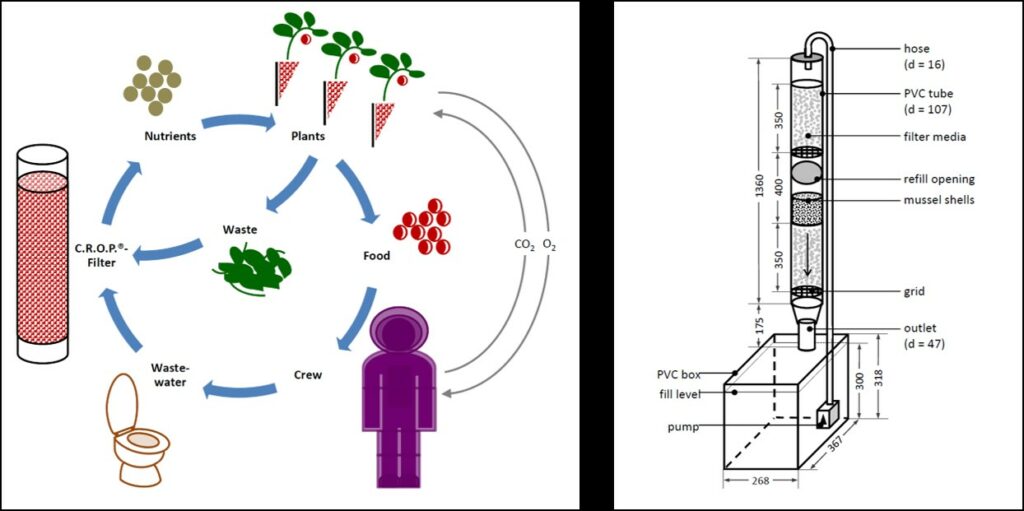
Schematic overview of the CROP® cycle
European Space Agency interns are focusing on DLR’s CROP® project, which aims to combine the decomposition of organic waste with a hydroponic system for growing vegetables. This system does not use soil and the roots of the plants are anchored in an artificial substrate or dangle directly in a nutrient solution. This nutrient solution is generated by a biofilter in which microorganisms process biological waste such as urea or food residues and can be used as fertiliser for growing vegetables without soil. Since all decomposition processes take place in an aqueous environment, the filter can be called a liquid compost heap[38] .
In the context of long space expeditions, it makes sense for the crew to have access to fresh food. A greenhouse system that produces fresh food on site is necessary to have a continuous supply of food without having to transport supplies from Earth to the Moon or Mars, and there are many projects dedicated to this. For several years on the ISS, astronauts have used equipment such as the European Modular Cultivation System (EMCS), launched in 2006, to study the growth of plants such as watercress. In 2018, the ECMS was replaced by a similar machine called the Biolab, but these projects were not focused on providing the astronauts with food, but rather on researching plant growth in Earth orbit and demonstrating the feasibility of space agriculture.
The EU-funded EDEN ISS project is building a real planetary greenhouse based on the analogue prototype ‘Mobile Test Facility’, which has been tested in Antarctica since February 2018. The greenhouse modules are separate and distinct elements from the habitat, but can be incorporated into the base’s life-support system, allowing it not only to grow food, but also to help revitalise the air and purify water. But no matter how detailed a project is, it will always be part of a larger infrastructure and it is necessary to understand what mission architecture it will be integrated into, and no such infrastructure/architecture currently exists. Furthermore, the microbiological environment and how plants will react to the spacecraft system with its special environment and contaminants are still unknown[39] .
A test of strength
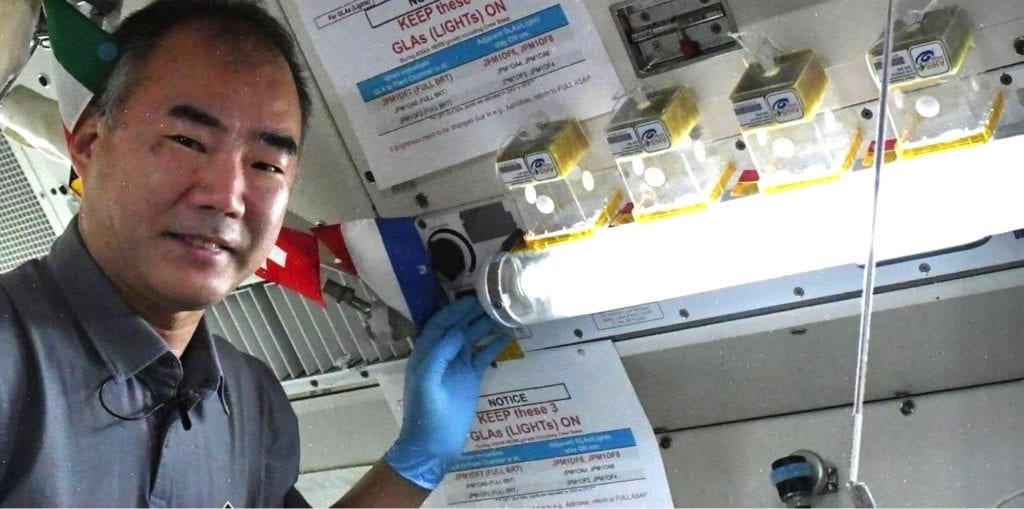
First shoots on the International Space Station[40]
Imagine yourself in a sort of bubble of atmosphere inhabited in the company of other strangers, where every day you perform a series of monotonous actions lasting more than two years. It sounds like the prologue to a psychological thriller. But if you add to this fantasy the physiological changes associated with gravity and isolation, exposure to radiation and alteration of the circadian rhythm, plus a sense of enormous risk: we are looking at the perspective of astronauts going to Mars.
NASA conducts a rigorous selection process for astronauts: only 60 candidates out of more than 18,000 will be allowed to go into space. Many candidates come from high-risk and high-responsibility fields (fighter pilots, doctors). Self-control and the ability to make ‘cold’ decisions are crucial. And even after countless psychological tests and checks, more than 50 per cent of medical incidents among astronauts on the ISS, so close to Earth, have been linked to psychological problems[41] .
In view of the ambitions of the next manned space missions and based on research and experience gained on the ISS and suborbital missions, as well as simulation studies and observations in near-earth conditions, the European Space Agency and its partners prepared a white paper, developed by independent European experts, reflecting unfulfilled research gaps related to the psychology of space exploration.
The paper addresses the issues of adaptation, teamwork, experiences before, during and after the mission, critical markers of psychophysical states, stress factors, individual and team characteristics and possible countermeasures to be developed and tested[42] . But it is fair to say that the issues to be addressed are many more than the preventive measures developed. It is difficult to predict and find a solution to a problem that no one has ever faced before, especially when dealing with a system as delicate and complex as the human psyche. There can be no models; people are not robots.
Influence of microgravity on human physiology
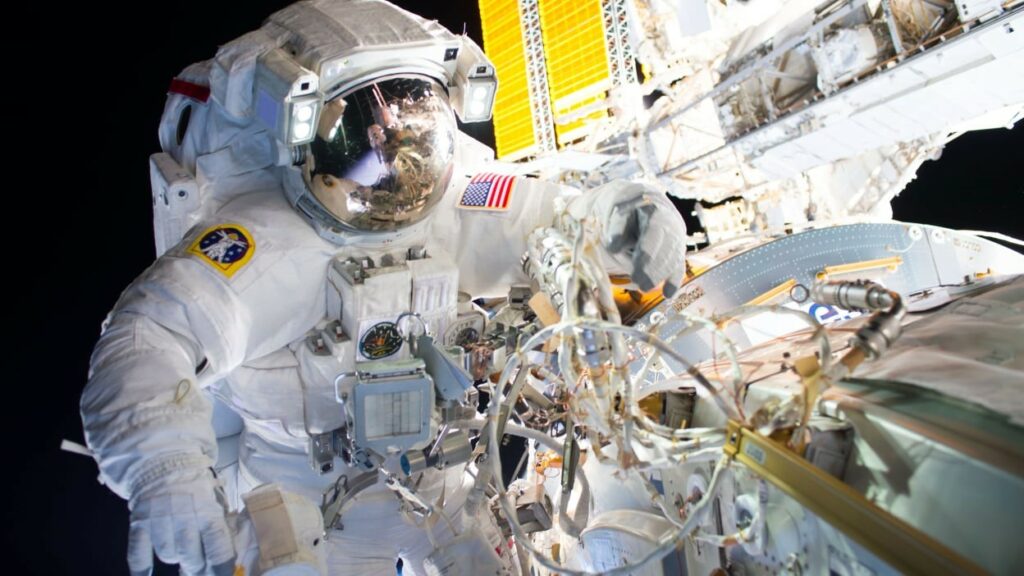
Scott Kelly, the American astronaut on whom the most advanced studies of brain damage caused by life in space have been done[43]
Humans are the most adaptable species on the planet, able to live in environments very different from Earth. But space presents a real challenge to human adaptability. Since humans evolved in Earth’s gravity and our entire body functions according to its laws, the absence of gravitational forces takes a heavy toll on all body systems. Despite our body’s ability to adapt to any condition and changing gravitational environment, it can have pathological consequences.
On Earth, the cardiovascular system works against gravity to prevent blood from collecting in the lower extremities, while microgravity causes a drastic redistribution of fluids from the legs to the upper body, dramatically increasing intracranial pressure. Over the course of weeks and months, plasma volume, red blood cell count and cardiac output decrease due to the reduced demands on the cardiovascular system to counteract gravity. When the astronaut returns to Earth, the low blood volume is not sufficient to maintain cerebral blood flow in an orthostatic position.
The effects of space on blood circulation occur in the following pattern: a) normal gravity (Earth); b) acute exposure to weightlessness (first 24 hours in space); c) prolonged exposure to weightlessness; d) return to Earth. The musculoskeletal system of an astronaut is in almost the same position as that of a bedridden patient; microgravity leads to profound muscular atrophy, the percentage of muscle mass loss can reach 50%.
Unfortunately, this problem is not always completely resolved after returning to Earth and many astronauts have problems with basic motor functions and with simply keeping the body upright. The stress on the bones is greatly reduced in space and the entire structure suffers, leading to skeletal demineralisation and reduced bone density. Calcium and other bone minerals are excreted with urine in greater quantities, so the risk of fractures increases.
In addition, space flight affects almost all parts of the brain to some extent[44] . Recently, an interesting experiment was conducted in which experts monitored the health of twins, one of whom, astronaut Scott Kelly, was on the ISS and his brother on Earth. One of the results was that Scott had shortened telomeres, the end sections of chromosomes that protect them from damage. Usually, a reduction in the length of these parts of the chromosomes occurs in the ageing process. In orbit, this effect is probably caused by increased background radiation[45] .
The effects of long-term space travel on an astronaut can be very severe and this calls for new disciplines that can address human adaptation to conditions we are not meant to endure. Frequent exercise, proper nutrition, an integrated network of biosensors capable of early detection of health markers, and drug therapies to support all vulnerable body systems are strategies used to combat the effects of space travel, but some changes in physical conditions are unavoidable and irreversible, and creating artificial gravity on a spacecraft or base, given the laws of physics we know today, is almost impossible.
The invisible danger

On Earth, life is protected from ionising radiation by the atmosphere and a magnetic field, which also protects the astronauts of the International Space Station, but despite this they receive during the day a radiation dose equivalent to that on Earth for a year[46] . The standard radiation dose for a human being on Earth is about 0.0036 Sv/year (0.36 rad); the Apollo astronauts, during their short mission of no more than 12 days, received an average radiation dose to the skin of 0.38 rad (the highest recorded dose was 1.14 rad) – which is comparable to two CT scans of the head[47] . The daily radiation dose on the lunar surface will be significantly higher, especially considering the duration of the mission, and cannot be quantified from Earth.
Based on data obtained by the Neutron and Dosimetry Research Facility (LND) aboard the Chinese lunar lander Chang’e 4 on radiation levels on the lunar surface, scientists estimate that astronauts in space suits will be exposed to about 60 microsieverts of radiation every hour. Overall, radiation levels could be 150 times higher than on Earth[48] . Exposure to radiation carries unpredictable dangers and can lead to various effects such as damage to the nervous system, acute/chronic radiation sickness, altered DNA structure, increased risk of cancer, tissue degeneration and more[49] . Despite the great progress made in 50 years of intensive research, the assessment of radiation risk and damage remains a complex topic, especially because the effects of radiation exposure depend on many factors and have a cumulative effect.
To address this problem, scientists are developing and testing waistcoats that protect astronauts during long missions, launching the epoxy resin Artemis I dummies, which replicate the bones, soft tissues and organs of an adult woman and are equipped with an incredible number of sensors and transducers. In order to measure and test the effects of radiation on women’s internal organs, the first manned mission to the Moon involves sending a female astronaut and biological experiments are being conducted in deep space with baker’s yeast (Saccharomyces cerevisiae), which is a near-perfect analogue of human genes, to determine how radiation affects the structure of DNA[50] .
But at the moment, scientists do not yet have enough experience with radiation from deep space. Not yet. As for options to deal with the problem locally, radioactively charged particles can be counteracted by building a particle shield of equal size. Hydrogen is the best option, better than steel or lead. Engineers are exploring the possibility of wrapping habitats in hydrogen-rich shells or creating inflatable structures and spacesuits using hydrogenated nanotubes.
A simpler option would be lunar soil, whose thick layer could provide protection from cosmic rays. Perhaps, if we combined the idea of building a structure with 3D-printed bricks made of lunar soil, filling it with regolith and inserting an inflatable habitat inside, we could protect ourselves not only from cosmic radiation, but also from the constant bombardment of micrometeorites to which the lunar surface is exposed[51] . At present, the meteorite threat is dealt with through observation from Earth and risk prediction[52] . On the ground, however, everything depends on chance.
Moon dust can also be a problem. Due to the lack of wind, its particles do not rub against each other and retain sharp edges; it also has an electrical charge and is attracted to literally everything. Lunar habitats must have special filters to keep it out, but outside it can be a problem for moving systems and mechanisms (antennas, solar panels, etc.). It can also be an allergen, as the astronauts of the Apollo missions found, even though they spent little time on our satellite[53] . Studying the properties of lunar dust is problematic because its behaviour on Earth and on the Moon is different. The intended use is also different: instead of using real moon dust for such important future research, it is being auctioned off[54] .
Houston, we are in trouble

The ESA Lunar Base Project[55]
The distance between the Earth and the Moon is about 384,400 kilometres and two-way radio communication can cover this distance in about 2.6 seconds[56] , while flight time takes about three days[57] . This means that, in the event of an emergency, it is possible to send a distress signal fairly quickly, get a response and hope that the rescue mission will be successful.
But the Moon in the context of colonisation is only a ‘stopping point’, all plans are directed towards Mars, whose minimum distance is 55.76 million km (when the Earth is exactly between the Sun and Mars) and maximum distance is 401 million km (when the Sun is exactly between the Earth and Mars)[58] . A one-way trip to the Red Planet will take about eight or nine months, while the round trip will take about 21 months, due to waiting for the favourable position of both planets[59] . When the mission arrives at Mars, Earth signals will take as long as 20 minutes to reach it. Add to this the time to compose a reply and send it, and you have a delay of at least 40 minutes[60] .
This makes communication inappropriate and implies a high level of autonomy that will be found in future missions, which means that the crew will have more responsibility for self-service and self-management. In the case of manned missions in deep space, the option of rescue missions is not considered. New strategies are needed for self-coping and self-management of health and performance that individuals and crews can use to maintain their functions[61] .
In addition, highly reliable systems are crucial when the remote crew does not have the possibility of being resupplied from Earth, as in the case of the space station. Small systems are also crucial and must function reliably to support life in space, from a functioning toilet to an automated fire-fighting system or exercise equipment to help astronauts stay fit[62] . There are no unimportant details in this field.
Thanks to international cooperation, continuous developments and technological advances, the dream of colonising other celestial bodies is approaching reality. But despite astounding technological advances in space exploration, enormous challenges loom on the horizon. Human space exploration tests the human adaptive response to a hostile environment where cosmic radiation, microgravity, physical confinement, vacuum and altered magnetic fields combine to threaten health with risks we do not expect.
The whole paradox of the adventure called ‘colonisation’ is that it is driven by the desire for knowledge, enhances the power of human thought, the brilliance of the idea and its technical realisation, and at the same time makes a human being a guinea pig. When we think about the boundaries of space, we must remain ambitious and curious, but also thoughtful, ethical and aware. Perhaps our journeys of many millions of kilometres can remind us how lucky we are to have a world of our own.
USA028
[1] https://www.engadget.com/nasa-artemis-program-explained-moon-mars-colonization-video-143013129.html
[2] https://www.nasa.gov/what-is-artemis
[3] https://www.nasa.gov/centers/marshall/artemis.html
[4] https://www.bbc.com/future/article/20230317-the-epic-quest-to-build-a-permanent-moon-base
[5] https://www.nasa.gov/gateway/overview
[6] https://www.bbc.com/future/article/20230317-the-epic-quest-to-build-a-permanent-moon-base
[7] https://www.engadget.com/nasa-artemis-program-explained-moon-mars-colonization-video-143013129.html
[8] https://www.humanmars.net/2021/01/steel-factory-on-mars-by-dmitry-ustinov.html
[9] https://www.nasa.gov/gateway/overview
[10] https://www.dailysabah.com/life/science/colonizing-the-moon-nasas-plans-for-lunar-base-under-artemis
[11] https://www.lockheedmartin.com/en-us/news/features/2022/bursting-the-bubble-with-inflatable-habitats.html
[12] https://arc.aiaa.org/doi/10.2514/6.2007-345
[13] https://www.sciencedirect.com/science/article/abs/pii/S027311772100805X
[14] https://interestingengineering.com/innovation/space-cement-is-here-how-it-could-be-used-to-build-houses-on-mars-and-the-moon
[15] https://www.degruyter.com/document/doi/10.1515/eng-2022-0390/html
[16] https://www.manchester.ac.uk/discover/news/scientists-develop-a-cosmic-concrete-that-is-twice-as-strong-as-regular-concrete/
[17] https://www.lockheedmartin.com/en-us/news/features/2022/bursting-the-bubble-with-inflatable-habitats.html
[18] https://www.engadget.com/nasa-artemis-program-explained-moon-mars-colonization-video-143013129.html
[19] https://www.dailysabah.com/life/science/colonizing-the-moon-nasas-plans-for-lunar-base-under-artemis
[20] https://redwirespace.com/newsroom/redwires-roll-out-solar-arrays-to-enable-lunar-power-infrastructure-for-astrobotic-vsat-program/
[21] https://hi-news.ru/technology/kak-dobyvayut-kislorod-v-kosmose.html
[22] https://www.skyatnightmagazine.com/space-science/how-long-does-take-get-space
[23] https://www.nasa.gov/feature/top-five-technologies-needed-for-a-spacecraft-to-survive-deep-space
[24] https://www.jpl.nasa.gov/news/nasas-perseverance-mars-rover-extracts-first-oxygen-from-red-planet
[25] https://www.sciencedirect.com/science/article/abs/pii/S0094576520301168?via%3Dihub
[26] https://www.space.com/perseverance-mars-rover-ends-moxie-oxygen-experiment
[27] https://oxeonenergy.com/moxie
[28] https://spacesettlementprogress.com/tag/isru/
[29] https://spacesettlementprogress.com/tag/isru/
[30] https://www.nature.com/articles/s41467-023-38676-2#Sec2
[31] https://www.space.com/chandrayaan-3-moon-south-pole-why-nasa-wants-to-go-too
[32] https://www.youtube.com/watch?v=qYW4rTrAA5I
[33] https://3dnews.ru/1084128/otkritie-kitayskih-uchyonih-dayot-nadegdu-na-dostup-k-sotnyam-milliardov-tonn-vodi-na-lune
[34] https://www.asc-csa.gc.ca/eng/astronomy/moon-exploration/viper-rover-mapping-water-ice-on-moon.asp
[35] https://www.space.com/viper-nasa-moon-rover-launch-delayed-2023.html
[36] https://nerdist.com/article/nasas-viper-rover-will-look-for-water-on-the-moon-south-pole-nobile-crater-interview/
[37] https://ec.europa.eu/research-and-innovation/en/horizon-magazine/food-and-water-systems-astronauts-will-need-travel-places-mars
[38] https://blogs.esa.int/exploration/spaceship-eac-recycling-water-on-the-moon/
[39] https://link.springer.com/article/10.1007/s12567-020-00318-4#Sec6
[40] https://www.dire.it/23-02-2021/606155-il-basilico-ora-cresce-anche-nello-spazio-e-germogliato-sulla-stazione-spaziale-internazionale/
[41] https://www.engadget.com/2019-07-19-nasa-astronaut-health-deep-space-missions.html
[42] https://www.ncbi.nlm.nih.gov/pmc/articles/PMC10063669/
[43] https://mashable.com/article/scott-kelly-record-broken-space-station
[44] https://www.ncbi.nlm.nih.gov/pmc/articles/PMC4206847/ ; https://sitn.hms.harvard.edu/flash/2013/space-human-body/
[45] https://universemagazine.com/ru/pochemu-cheloveku-budet-tyazhelo-na-lune-fiziologicheskie-problemy-vnezemnoj-zhizni/
[46] https://universemagazine.com/ru/pochemu-cheloveku-budet-tyazhelo-na-lune-fiziologicheskie-problemy-vnezemnoj-zhizni/
[47] https://history.nasa.gov/SP-368/s2ch3.htm
[48] https://link.springer.com/article/10.1007/s11214-020-00725-3
[49] https://www.sciencedirect.com/science/article/pii/S0094576523003727
[50] https://www.smithsonianmag.com/science-nature/how-space-radiation-threatens-lunar-exploration-180981415/
[51] https://www.iop.org/explore-physics/moon/how-could-we-live-on-the-moon#gref
[52] https://www.nasa.gov/centers/marshall/news/lunar/overview.html
[53] https://universemagazine.com/ru/pochemu-cheloveku-budet-tyazhelo-na-lune-fiziologicheskie-problemy-vnezemnoj-zhizni/
[54] https://universemagazine.com/ru/lunnaya-pyl-s-apollo-11-za-400-tysyach-dollarov-obladaet-otvratitelnym-sekretom/
[55] https://www.esa.int/Space_in_Member_States/Italy/ESA_testa_la_stampa_in_3D_per_costruire_una_base_lunare_con_l_aiuto_di_una_tecnologia_italiana
[56] https://www.wionews.com/india-news/how-will-communication-link-between-chandrrayaan-2-orbiter-and-chandrayaan-3-lander-help-isro-627585
[57] https://www.techinsider.ru/technologies/1567219-skolko-vremeni-letet-do-luny-v-budushchem-vam-prigoditsya-eto-znanie/
[58] https://mapgroup.com.ua/articles/bezgranichnyj-kosmos/775-rasstoyanie-ot-zemli-do-marsa-skolko-vremeni-letet-do-marsa
[59] https://www.space.com/24701-how-long-does-it-take-to-get-to-mars.html
[60] https://www.engadget.com/2019-07-19-nasa-astronaut-health-deep-space-missions.html
[61] https://www.ncbi.nlm.nih.gov/pmc/articles/PMC10063669/
[62] https://www.nasa.gov/feature/top-five-technologies-needed-for-a-spacecraft-to-survive-deep-space
Leave a Reply